Article of the Month -
November 2005
|
Hydrography and Disaster Management
Adam J. KERR, United Kingdom
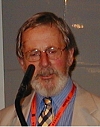
This article in .pdf-format
1)
This paper has been for the first time presented at the FIG Forum at the
Intergeo 2005 in Düsseldorf, Germany, 4 October 2005.
1. INTRODUCTION
In recent years several major disasters have occurred involving the
worldwide coastal area. The most recent has been hurricane Katrina and its
disastrous effects on the city of New Orleans. On December 26, 2004 there
was the major Asian tsunami that seriously affected the countries around the
Indian Ocean and in which nearly 300,000 people died. Earthquakes and their
sometimes associated tsunamis are a relatively frequent event in many parts
of the world that are subject to seismic activity. A short list of some
recent major tsunami events is provided by the USA NOAA Environmental
Laboratory (www.pmel.noaa.gov/tsunami/database_devel.html)
as follows:
- March 28, 2005 Indonesia
- December 26, 2004 Indonesia (Sumatra)
- September 25, 2003 Hokkaido
- June 23, 2001 Peru
- January 13, 2001 El Salvador
- November 26, 1999 Vanuatu
- July 17, 1998 Papua New Guinea
Prior to the above two significant tsunamis took place at Prince William
Sound, Alaska in 1968 and Chile in 1960.
While major disasters in coastal areas are normally due to tsunamis or
hurricanes, other disasters may occur in the form of ship grounding and
major oil pollution and flooding caused by a combination of unusually heavy
precipitation, sometimes linked with abnormally high tides. Such an event
may be recalled when in 1956 a major storm caused extensive flooding in
several countries surrounding the southern North Sea. That resulted in a
major programme of public works, with dykes built in the Netherlands and the
Thames Flood Barrier being built on the Thames in 1986 to prevent flooding
in the city of London, at a cost of £ 370 million.
While the response to these disasters is initially in the hands of
emergency measures personnel, such as firemen, policemen and medical staff,
the longer term activity, directed at their future prediction and ideally
their prevention, falls more into the hands of scientists and engineers with
a great variety of backgrounds. Whether the forces causing the disasters are
meteorological or geological there is an urgent need to be able to predict
more precisely just when and where they will occur. When they do occur we
need to be able to predict the locations where remedial measures should be
taken to minimise the damage to property and human beings. It is plainly
evident that in most cases the worst damage occurs to low lying flat areas
and these unfortunately are where it has been found most convenient and
economic to build our urban areas. Political response sometimes results in
engineering studies and major public works to minimize future destruction.
No doubt, this will be one of the outcomes of studies following the Katrina
hurricane disaster.
Hydrography has an important part to play in both prediction and
response, although in most cases, these activities will take place as part
of multi-disciplinary teams. Basic mapping of several parameters is
fundamental to successful modelling and prediction. Fortunately, in recent
years new instruments have become available, which allow hydrographers to
carry out their work more quickly and thoroughly and instruments such as
multibeam acoustic systems and airborne laser systems (LIDAR) provide very
effective tools for the task.
2. HYDROGRAPHY IN PREDICTION
Seismic disturbances in or close to the marine areas are the cause of
most tsunamis. While seismic disturbances occur in all of the world’s
oceans, the Pacific Ocean, which is surrounded by a ring of volcanic
activity, and mainly due to the subduction of tectonic plates, has long been
recognised as an area where tsunamis occur frequently. It has been noted
that the number of significant tsunamis in the Indian Ocean, the site of the
disastrous 2004 tsunami, is less than a dozen in the last 100 years,
compared with the one hundred or more in the Pacific Ocean during the same
period. (Gupta H.K.,2005). The actual study of seismic activity in a general
sense is clearly in the hands of geologists and seismologists. In countries
such as Japan, their research is fundamental to the economic prosperity as
well as the safety of the people. The translation of this seismic activity
into tsunami distribution and propagation involves work of a more
multi-disciplinary nature. Once the seismic activity has taken place on the
seafloor, sub-seafloor or nearby land we need to determine just how the
tsunami will propagate in terms of direction and speed. We will also need to
predict such matters as wave length and amplitude as it approaches a
vulnerable coast. Here hydrographers have a role, as has been shown recently
in the surveys carried out by the UK Hydrographic Office, aboard HMS Scott,
in the waters off Sumatra. (UKHO, 2005) Using a mulltibeam acoustic echo
sounder (MBES) the surveyors were able to accurately map an area of the
seafloor in the vicinity of the epicentre of the seismic disturbance, that
caused the major Asian tsunami on December 26, 2004. Working together with
geologists and geophysicists, who used seismic instruments and conducted
coring, they were able to provide information that permitted a better
understanding of the trigger that set off the disturbance. While this type
of research is undoubtedly helpful in making future predictions, the
difficulty is in the immensity of the task. Huge ocean areas where
subduction occurs represent an overwhelming survey task. Even with the
advantages of MBES the precise systematic surveying of the oceans is a huge
task. It has been noted (Yeh,1995) that for tsunami computations the
resolution of depth measurements on a grid finer than around 2 km. may not
be necessary in water deeper than 2000 m. but if there is a narrow canyon or
ridge about 2 km. wide in water of 1000 m. a higher resolution of about 500
m. spacing of the depth data will be needed. Other ocean survey tasks demand
the interest and limited resources of the hydrographers and submarine
geologists. The best that can be done at present is to obtain sufficient
data for generalised modelling and to understand the basic mechanisms which
set off a tsunami.
Most tsunamis are caused by a rapid vertical movement along a break in
the earth’s crust. Typically this mechanism only occurs in large subduction
zones. Subduction occurs around most of the Pacific, with the exception of
the western coast of North America, where movement along the faults is
largely strike-slip (Lander, Lockbridge and Chinnery, 1989).
While most current research is directed at seismicity as the trigger
mechanism, some research is investigating the possibilities of landslides
and underwater slippage. This has caused locally destructive tsunamis in
Alaska, being the subsequent cause of considerable re-charting of the area.
Research has also been carried out in recent years by the National
Oceanographic Centre in the UK, which has been examining such possibilities
in the Canary Islands. The importance of obtaining detailed bathymetry has
been made clear in this work (Wynn, 2003). Limited earlier bathymetry did
not show that there was slumping and landslides but more recent detailed
surveys have shown these features. From this it may be speculated that a
trigger mechanism for tsunamis does exist in this area of the Atlantic
Ocean. While media reports have tended to exaggerate this danger it is seen
as less critical by scientists but nevertheless provides an interesting
model to study the possible mechanism of tsunamis caused by landslides.(www.noc.soton.ac.uk/CHD/Research/topics/canaries_slides.html)
3. SEA LEVEL MONITORING
An important means of monitoring and predicting tsunami activity is in
the monitoring of sea level change around an ocean area. (Rabinovich and
Stephenson, 2004) This has been a prime focus of activity for the countries
bordering the Pacific for many years. An International Tsunami Warning
System for the Pacific was initially established at Honolulu, Hawaii in
1946, and the present organisation came into being in 1968. Its task is to
co-ordinate the national monitoring activities, and with a knowledge of
tsunami characteristics and tools such as tsunami models and historical
tsunami databases, to advise countries of the possible occurrence of a
tsunami. Water level gauges operated by most hydrographic offices, whose
main task is to provide data for the analysis and prediction of tides, can
provide this secondary important task of detecting anomalies in the form of
a change of water level height caused by a tsunami. Research in some of the
national offices that are involved in this enterprise includes the difficult
task of distinguishing the seismically induced long waves from those
produced by meteorological forces, termed seiche oscillations. In recent
years, and it may be noted, prior to the 2004 Asian tsunami, the network and
technology of the water level monitoring stations was considerably improved.
Advantage has been taken of the availability of digital measuring devices
which provide much higher precision than the earlier analogue devices. These
record water level heights at one minute intervals. Modern satellite
communications contribute immeasurably to the networking of the information
and monitoring the progression of the tsunami waves. The monitoring of
tsunamis not only for providing advice and warning when damaging tsunamis
are likely to occur, but also if they will not cause damage, as the
mechanism of false alarms is most important.
4. OFFSHORE MONITORING USING BUOYS
Measurement of sea level heights in the open ocean by means of buoys,
connected to pressure sensors located on the sea floor, today supplement the
terrestrially based, water level observations along the shoreline. As part
of the US National Tsunami Mitigation Program deep ocean tsunameters have
been developed and are now distributed across parts of the Pacific Ocean
under a programme called DART (Deep-ocean Assessment and Reporting of
Tsunamis) (www.pmel.noaa.gov/tsunami/Dart/dart_pbl.html).
These devices are bottom mounted and transmit data acoustically to surface
buoys equipped with satellite transmitters. It may be noted that this
extension of the activity tends to take it out of the hands of the
hydrographic community. At a workshop held in Paris in March 2005, a
monitoring system for the Indian Ocean was discussed. It was suggested that
10 monitoring buoys should be commissioned for the Bay of Bengal without
delay (Johnson, 2005). The importance of using existing buoy networks, of
which there are several in the Indian Ocean, was emphasised at the meeting.
The study of the propagation of tsunamis across the deep oceans has been
a source of much scientific interest and has resulted in numerous papers
being written on modelling and forecasting of tsunami wave scattering ,e.g.
the MOST ( Method of Splitting Tsunamis) model discussed by Titov and
Gonzalez (Titov and Gonzalez, 1997) Essentially the propagation of the
tsunami wave is dependent upon the depth of the ocean but may be deflected
by changes in the sea floor topography. In deep ocean depths the tsunami
waves move at a speed of more than 1000 km. per hour and can cross oceans
within a day. Because the speed of the tsunami depends on the depth of the
ocean basins, the waves decrease in speed as they reach shallower water. The
wave length is shortened and the energy within each wave is crowded into
progressively less water, increasing the height of the Wave. (Lander,
Lockbridge and Chinnery, 1989). Depending upon the direction in which the
wave approaches the shallow water, either perpendicularly or obliquely, it
may be deflected in direction. In addition, offshore bathymetric features
such as seamounts or submerged ridges focus the wave energy and produce
extremely large waves at some coastal locations. The importance of this
knowledge was only too apparent in assessing the devastation caused by the
Asian tsunami. Generally speaking this area of research falls more to the
physicists and mathematician than to the hydrographer, although the depth
data that hydrographers collect and compile will be fundamental to the
modelling activity. Here again bathymetric knowledge is a pre-requisite for
the modelling. While the state of general knowledge of the ocean bathymetry
remains limited it may suffice given the overall limitations of present
models.
5. PROBLEMS OF THE COASTAL ZONE
It may be noted that most disasters occur in the coastal zone, the area
where land and sea meet. Undoubtedly a mariner meeting a hurricane in the
open sea may be fearful for his safety, but he needs to be much more so when
the hurricane, having gained all its strength from the warm sea, actually
collides with the land. From that point the hurricane will begin to lose its
strength, although it may cause problems of heavy rain and consequent
flooding for many miles inland. As noted above, the tsunami wave increases
in amplitude as it approaches shallow water and consequently it will be at
its most destructive as it reaches the shore, although other side effects
caused by the storm surge with its associated rise in sea level and
consequent flooding, may in the end cause just as much damage. As a means to
making risk assessment of potential damage caused by these events and as a
means to support action taken to recover from these events afterwards, basic
mapping of the coastal zone, in considerable detail is essential for
effective modelling (Yeh, 1995). Yeh has stated that a 100 m. depth grid is
needed for depths less than 10 m. and a 50 m. grid is needed where depths
are less than 2.5 m. Research on the process of inundation caused by
tsunamis has been carried out in the area of Juan de Juan Strait in
northwestern USA (Venturato et al, 2004) Detailed and accurate information
on both the wet and dry topography are fundamental to the success of the
models. Unfortunately, it has only been in recent years that any priority
has been given to mapping of the interface between land and sea.
Hydrographers have been mainly concerned with the area where ships float,
not where they do not. Land surveyors have often seen the mud flats, that
often border the dry land, as useless for development and consequently of
little interest to map. A great increase in environmental concern has caused
several hydrographic offices to address the surveys of the coastal zone with
more urgency. Hydrography has taken on a broader mandate of not just
surveying for navigational reasons but to provide data for a variety of
purposes. However administrative difficulties exist, such as national
copyright making it difficult for researchers to obtain the data and an
international pool of this inshore data has been suggested (Yeh, 1995).
Besides the administrative reasons for not giving more attention to the
coastal zone there have also been some technical reasons. Historically
hydrographers, in order to provide a margin of safety to navigators, have
used low water as a vertical datum to which all depth measurements were
reduced. Land surveyors, on the other hand have preferred to use mean sea
level as their vertical reference. These differences have resulted in a step
along the shoreline that has made it difficult to ensure a smooth transition
between the marine and land data. There is now considerable interest in
developing a common vertical reference frame, so that the mapping and
charting of land and sea can be seamless. (Parker, 2002). A particular
difficulty of surveying the coastal zone has been its hostility in many
places. Either there is heavy wave action and surf, making it difficult for
survey boats to work in the area or it may be large muddy flat areas, which
are also very difficult to survey. However help is at hand and there is now
increasing use of airborne LIDAR which allows large areas of coastal zone to
be surveyed precisely and rapidly. Such surveys have now been carried out
over extensive lengths of the coastline of the USA. (Lillycrop, 2005).
Interferometric sidescan or other forms of wide swath sonar also promise to
expedite shallow water bathymetry surveying.
Research has been carried out into the inundation of coastal areas as a
result of flooding by tsunamis (Venturato et al, 2004) and may also have an
application in flooding by hurricanes and other causes. Whether this
information will be heeded any more by city planners in the coastal zone
than it is for planners that still seem to allow houses to be built on flood
plains remains to be seen.
6. HYDROGRAPHY IN THE AFTERMATH
Institutionally, the aftermath of a disaster brings much hand ringing and
people expressing “what if!” It was noted earlier that in the first stages
much of the work falls on the emergency measures teams. However, these teams
must first reach the stricken areas and this will require a replacement of
the infrastructure. In the marine sense this means that ships carrying
relief supplies or even moving refugees, can enter the area. Immediately
after the Asian Tsunami disaster a number of maritime organisations, headed
by the International Maritime Organisation (IMO) met in London, on 12
January, to assess the action that had to be taken (IMO, 2005 ). This group
included the International Hydrographic Organisation (IHO). It was critical
that the promulgation of maritime safety information was updated and
dispatched as soon as possible. That task fell on the national hydrographic
offices. Major changes in the topography and particularly the existence of
objects that had been washed into the navigable channels, have to be
surveyed with dispatch. An action plan developed at the IMO meeting
contained the following elements:
Short term
- Assess the extent of damage to navigational aids in the affected
areas, in cooperation with the national authorities.
- Assess and undertake preliminary re-survey for any reported changes in
depths in the affected areas particularly ports, restricted navigational
areas, the Malacca Straits and other areas as needed.
- Issue advice to shipping as appropriate, through existing networks.
Medium/long term
- Technical co-operation activities including needs’ assessment
missions; mobilizing and co-ordinating resources accordingly
- Assess and define new charting requirements
- Marking of new dangers, if necessary
- Participate in establishing an appropriate tsunami early warning
system for the Indian Ocean, in co-operation with UNESCO/IOC and others
Consider and incorporate consequential amendments to the Organisation’s
basic documents as appropriate.
Regionally, UNESCO arranged a Workshop to discuss the development of a
Tsunami Warning and Mitigation System for the Indian Ocean within a Global
Network in 2005 (UNESCO, 2005 )
At a national level the US Government also set out a plan for an improved
tsunami detection and warning system. This included a promise of $ 37.5
million over the next two years to expand US detection and monitoring
capabilities. Its elements include much of the research touched on in this
paper.
The above type of work has taken a more direct and practical shape in the
aftermath of the hurricane Katrina disaster. The US Coast Survey of NOAA has
moved in directly with its Navigation Response Teams ( NRTs) to coordinate
the activities of several survey vessels working the area of New Orleans and
the coast of Louisana. (http://chartmaker.ncd.noaa.gov/nsd/katrina.htm).
This work will no doubt be carried out in close co-operation with other US
national agencies, such as the US Army Corps of Engineers, the US Naval
Oceanographic Office and the US Coast Guard. It has been noted that these
emergency services include performing side scan sonar surveying for updating
US Government Navigational Charts, conducting hazardous obstruction surveys
(utilizing diving operations), electronic navigation capture, data
collection and mapping support activities.
7. THE FUTURE
It is a certainty that other disasters will occur in the future.
Considerable political speculation exists on the extent and type of future
man-induced disasters but other major natural disasters remain inevitable.
Hydrographers have a part to play, both in their capacity as providers of
various types of marine data, particularly of the sea floor topography and
the tides, but also as part of multi-disciplinary teams that work towards
improving our ability to predict future maritime disasters, their
consequences and minimization of their effects. Fortunately, recent
developments in technology will aid them in their task. The broad and
general use of satellite positioning will permit a common and precise
geographic framework for any information. MBES permits sea floor mapping to
be carried out quickly and precisely, although it should be recognised that
when it comes to the oceans themselves the area still to be mapped is
daunting. LIDAR is being increasingly used, although it must be borne in
mind that it does not work well in turbid water and that is the normal
condition immediately following a disaster. Finally, due to advances in
telecommunications and information technology, our ability to manage data
has improved significantly in recent years.
It is typical following a disaster, be it a major shipwreck, a tsunami or
a hurricane, for the political process to put in place various systems to
try to alleviate the event happening again. Examples of this can be seen in
the requirement for all ships to continually man their wirelesses, following
the sinking of the Titanic or more recently, the development of a tsunami
warning system for the Indian Ocean. However it is essential that once the
political heat has passed, that ongoing support for marine science in its
broadest sense, continues to be provided, because it is from the sea that
most of these disasters develop.
8. CONCLUSIONS
Hydrography plays an important role in marine disaster management, both
in its prediction and in the aftermath. Increasingly marine sciences are
becoming multi-disciplinary and hydrography has an important part to play
both in the scientific research and in engineering work that may anticipate
or follow a disaster. Traditionally hydrographic surveys were mainly
directed towards providing data for the production and maintenance of
nautical charts but today hydrography has a much broader mandate and
provides data for a great range of environmental and engineering studies.
Basic mapping of the bathymetry is fundamental to monitoring tsunamis in
both the deep oceans and in the coastal zone. Modern technology in the form
of multi beam and interferometric systems are being employed to provide the
dense data sets for much of the modelling. The systematic measurement of
water levels in many countries provides an important method detecting
tsunami dynamics. The measuring stations are linked by communications to
provide important warning systems. To date this activity has been mainly
centered in the Pacific Ocean but the recent disastrous tsunami in the
Indian Oceans has brought about the political will to extend the network
internationally. It is important that such political will extends beyond the
period immediately after the disasters to provide ongoing funds of a
substantial nature for future scientific research into prediction and risk
analysis and to the construction of reliable engineering defences.
REFERENCES
- US Marine Environmental Laboratory. NOAA
www.pmel.noaa.gov/tsunami/database_devel.html
- UKHO (2005) Indian Ocean Tsunami Briefing.
- H.Yeh (1995) The Tsunami Perspective. IOC Technical Series No. 63 pp
45-47.
- H.Yeh (1998) Tsunami researchers outline steps for better data. EOS.
Trans.Amer.Geophys Union 79, 480 and 484.
- Gupta H./K.(2005) Early Warning Systems for Tsunamis and Storm Surges:
The Indian Initiative. UNESCO Workshop Report. No. 196.
- Wynn R.B. and Masson D.G. (2003) Canary Islands landslides and tsunami
general Symposium on Submarine mass movements and their consequences ( Ed.
By J.Mienert, Dortrecht, 325-332.
- Wynn R.B.(2003)
www.noc.soton.ac.uk/CHD/Research/topics/canaries_slides.html.
- Rabinovich A.B. and F.E.Stephenson (2004) Longwave measurement for the
coast of British Columbia and Improvements to Tsunami Warning capability.
Natural Hazards 32: 313-343.
- NOAA (2005) Deep Ocean Assessment and Reporting of Tsunamis. The DART
program.
http://www.pmel.noaa.gov/tsunami/DART/dart_pbl.html.Lander J.F.,
P.A.Lockbridge and M.A.Chinnery (1989) United States Tsunamis (including
US Possessions) 1690- 1988. pp.2-4.
- Titov V.V. and F.I.Gonzales ( 1997). Implementation and Testing of the
Method of Tsunami Splitting (MOST). NOAA Technical Memorandum ERL PMEL –
112. November.
- Parker B. (2002) The Integration of Bathymetry, Topography and
Shoreline and the Vertical Datum behind it. I H Review 3 # 3 November. pp
14-26.
- Lillycrop J. (2005) Status Report on the Joint Airborne LIDAR
Bathymetry Technical Center of Expertise and CHARTS First Year of
Operation. Proc. US Hydro 2005. March 29-31. San Diego, USA.
- Venturato A.J., V.V.Titov, H.Mojfeld and F.I.Gonzalez (2004) NOAA TIME
Eastern Strait of Juan de Fuca, Washington, Mapping Project, NOAA
Technical Memorandum OAR, MEL Contribution 2713.
- International Maritime Organisation ( 2005) IMO, IHO and IALA meet to
coordinate Tsunami Responses. Briefing 04/2005. 12 JanuaryUNESCO (2005)
International Coordination Meeting for the Development of a Tsunami
Warning and Mitigation System for the Indian Ocean within a Global
Framework. IOC Workshop Report No. 196 p 35.
BIOGRAPHY
The author has previously been a Director of the International
Hydrographic Organisation. He is now Editor of the International
Hydrographic Review.
CONTACTS
Adam Kerr
Flagstaff Cottage
Lamorna
Penzance
Cornwall TR19 6XQ
UNITED KINGDOM
Tel. + 44 1736 731 228
Fax + 44 1736 731 976
E-mail:
Adam.J.Kerr@btopenworld.com
 |